An Overview of Proteomics
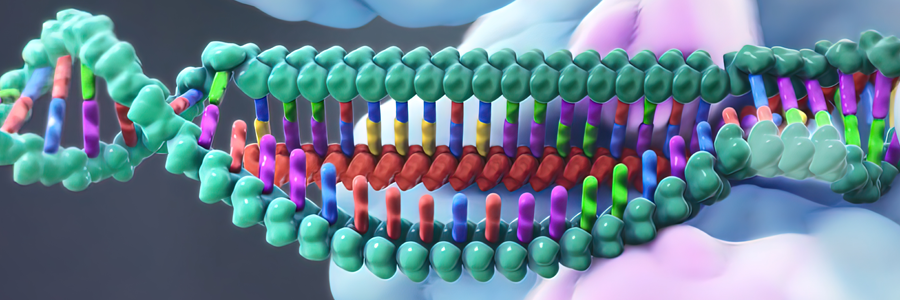
Proteomics is the study of proteins in a biological system that offers the required understanding of an organism’s structure and function compared to genomics. Proteins are the functional units of cells, performing various crucial tasks. This article will provide an overview of proteomics and how it analyzes their abundance, modifications, interactions and localization, providing insights into complex molecular events. We will also discuss how this field enhances our understanding of life’s intricacies, helps in the discovery of biomarkers and allows for the identification of therapeutic targets.
What is proteomics?
Proteomics is a field of scientific research that focuses on the comprehensive study of proteins, including their interactions, functions, compositions and structures within living organisms. It is an area of study that has gained significant importance in understanding various biological processes and the underlying mechanisms of diseases.
Proteins are essential molecules that perform various functions in living organisms. They play crucial roles in cellular processes such as metabolism, signaling and gene expression, and they are involved in maintaining the structure and integrity of cells and tissues. By studying proteins, scientists can gain insights into how they contribute to the overall functioning of an organism.
In proteomics, researchers aim to identify and characterize all the proteins in a given biological sample, such as a cell, tissue or body fluid. This involves techniques for protein separation, quantification and analysis. One common method used in proteomics is mass spectrometry, which helps identify and quantify proteins based on their mass and charge.
Additionally, proteomics involves studying protein-protein interactions and protein networks within cells. By understanding how proteins interact with each other, scientists can unravel complex signaling pathways and regulatory networks that govern cellular processes. This information can help in identifying potential drug targets and designing therapeutic interventions.
Furthermore, proteomics plays a significant role in personalized medicine and biomarker discovery. By comparing protein profiles between healthy and diseased individuals, scientists can identify specific proteins or patterns of proteins that are associated with certain diseases. These protein biomarkers can be used for detecting diseases early, monitoring treatment responses and developing personalized treatment strategies.
The importance of proteomics
Proteomics’ ability to uncover protein functions, understand disease mechanisms and advance personalized medicine and diagnostics makes it an indispensable tool for improving human health and advancing our knowledge of biology. Its significance can be attributed to several key aspects:
- Revealing Protein Functions
Proteins are the workhorses of biological systems, carrying out vital functions in cells and organisms. Proteomics allows researchers to identify and characterize the functions of proteins, providing insights into their roles in different biological processes. By understanding protein functions, scientists can unravel complex cellular pathways, discover novel therapeutic targets and develop more effective drugs.
- Understanding Disease Mechanisms
Many diseases, including cancer, neurodegenerative disorders and cardiovascular diseases, are associated with protein expression, structure or function alterations. Proteomics enables the study of these disease-related changes at a global scale. By comparing protein profiles between healthy and diseased individuals, researchers can identify disease-specific biomarkers and gain insights into the underlying mechanisms. This knowledge can help in early detection, personalized treatment and the development of targeted therapies.
- Advancing Personalized Medicine and Diagnostics
Proteomics has the potential to revolutionize personalized medicine by enabling precise and tailored treatment strategies. By analyzing protein profiles, researchers can identify biomarkers that are indicative of a patient’s response to specific treatments or predict disease outcomes. This information can help physicians select the most effective therapies and avoid unnecessary treatments, improving patient outcomes and reducing healthcare costs.
- Drug Discovery and Development
Proteomics plays a vital role in drug discovery by providing valuable information about drug targets and mechanisms of action. By studying protein-protein interactions and protein networks, scientists can identify key molecular targets for therapeutic intervention. Proteomics also helps assess drug efficacy and safety, enabling the development of safer and more effective drugs.
Types of proteomics
Proteomics encompasses three main types: expression proteomics, structural proteomics and functional proteomics. These three types of proteomics together offer a comprehensive approach to studying proteins, covering their expression, structure and functional characteristics.
Expression proteomics focuses on identifying and quantifying proteins in a sample, providing valuable insights into biomarker discovery and cellular responses. Structural proteomics, on the other hand, aims to determine the three-dimensional structures of proteins using techniques like X-ray crystallography and nuclear magnetic resonance (NMR) spectroscopy, enabling researchers to explore the intricate architecture of proteins. Lastly, functional proteomics investigates the roles and interactions of proteins, shedding light on their biological functions.
By encompassing these three types, proteomics provides a multifaceted approach to unraveling the complexities of proteins in various biological processes.
Expression Proteomics
Expression proteomics focuses on studying the quantitative changes in protein expression levels in response to different biological conditions or stimuli. Techniques such as two-dimensional gel electrophoresis and quantitative mass spectrometry are commonly used for protein quantification in expression proteomics. Additionally, stable isotope labeling methods and label-free approaches allow for comparative protein expression analysis across samples. Expression proteomics has significant applications in understanding disease mechanisms, as it can reveal protein alterations associated with diseases and identify potential therapeutic targets.
Structural Proteomics
Structural proteomics aims to determine the three-dimensional structures of proteins and their complexes. Techniques such as X-ray crystallography, NMR spectroscopy and cryo-electron microscopy (cryo-EM) are commonly employed in structural proteomics. By determining protein structures, researchers can gain insights into protein function, interactions and drug design. Structural proteomics is essential to understand protein-protein interactions, enzyme mechanisms and ligand binding sites. Computational approaches are also used to predict protein structures and model protein-protein interactions. Structural proteomics has broad applications in drug discovery, as it aids in identifying drug targets and designing molecules that can selectively bind to specific protein structures.
Functional Proteomics
Functional proteomics focuses on understanding the functional roles of proteins in biological processes. It employs various techniques to study protein-protein interactions, post-translational modifications (PTMs) and protein localization. Methods like yeast two-hybrid (Y2H) assays and protein microarrays are used for protein-protein interaction studies, providing insights into protein networks and signaling pathways. Analysis of PTMs, such as phosphorylation and acetylation, helps elucidate regulatory mechanisms and protein activity. Protein localization studies help understand protein distribution within cells and their involvement in cellular processes.
Basic proteomics workflow
The proteomics workflow involves key steps for comprehensive protein analysis. Sample fractionation separates complex protein mixtures into smaller subsets, reducing complexity and enriching specific protein fractions. Enzymatic digestion, typically using trypsin, breaks proteins into peptides for analysis. Peptide extraction is performed, followed by mass spectrometry analysis to identify and characterize the peptides, providing insights into the protein composition and structure.
Sample Preparation and Fractionation
Sample preparation is the initial step in proteomic workflows that involves techniques for sample lysis, protein extraction and fractionation methods to reduce sample complexity and enrich target proteins for analysis. Techniques like gel electrophoresis, liquid chromatography or solid-phase extraction are commonly used for protein fractionation. Sample preparation ensures the efficient extraction of proteins from complex biological matrices, removes interfering substances and enhances the detection of low-abundance proteins. Proper sample preparation is essential for obtaining reliable and reproducible proteomic data and plays a pivotal role in downstream analysis.
Enzymatic Digestion
Enzymatic digestion is a key step in proteomic workflows, typically using the protease trypsin. It involves the cleavage of proteins into peptides for subsequent analysis. The selection and optimization of digestion conditions are crucial to ensure efficient and specific protein cleavage. Factors like digestion time, temperature, enzyme-to-protein ratio and digestion buffer composition must be optimized. Digestion controls are essential for monitoring digestion efficiency and reproducibility across samples. Efficient enzymatic digestion enables the generation of peptide fragments suitable for identification and quantification in downstream mass spectrometry analysis.
Peptide Extraction and Cleanup
Peptide extraction and cleanup are essential steps following enzymatic digestion in proteomic workflows. Various extraction methods, such as solid-phase extraction, liquid-liquid extraction or precipitation, are employed to isolate peptides from the digestion mixture. These methods help remove contaminants, salts and detergents that may interfere with downstream analysis. Peptide cleanup techniques further enhance sensitivity and reduce interference during mass spectrometry analysis. Cleanup methods may involve desalting, removing small molecules or eliminating residual reagents. Proper peptide extraction and cleanup improve the quality of the peptide samples, leading to more accurate and reliable proteomic analysis results.
Mass Spectrometry Analysis
Mass spectrometry (MS) analysis is another important step in proteomic workflows that help identify peptides and proteins. It involves ionization techniques such as electrospray ionization (ESI) and matrix-assisted laser desorption/ionization (MALDI) to generate gas-phase ions from peptides. Mass analyzers, including time-of-flight (TOF), quadrupole and Orbitrap, separate and measure the mass-to-charge ratio (m/z) of ions. Data-dependent acquisition (DDA) strategies help select the precursor ions for fragmentation, facilitating peptide identification and quantification. Data analysis strategies involve database searching, matching observed spectra with theoretical peptide sequences, and software tools for protein identification, quantification and data interpretation in MS-based proteomics.
Challenges of proteomics
Various proteomics challenges include sample complexity, data analysis and interpretation, standardization and reproducibility, dynamic range and sensitivity, post-translational modifications, and integration of multiple omics data. Biological samples contain diverse proteins with varying abundances, requiring advanced fractionation techniques for comprehensive analysis.
Sample Complexity
Sample complexity is a significant challenge in proteomics due to the vast array of proteins present in biological samples. The wide dynamic range of protein abundance poses difficulties, as high-abundance proteins can hinder the detection of low-abundance ones. To overcome this challenge, effective sample fractionation and enrichment strategies are employed. Fractionation techniques such as gel electrophoresis, liquid chromatography or solid-phase extraction help reduce sample complexity by separating proteins based on different properties. Enrichment methods, such as antibody-based affinity capture, target specific protein subsets for in-depth analysis.
Data Analysis and Interpretation
Data analysis and interpretation pose significant challenges in proteomics due to the large volumes of data generated and the complexity of protein identification and quantification. Analyzing high-throughput proteomic datasets requires robust bioinformatics tools and databases for data processing, analysis and result interpretation. Protein identification involves matching experimental spectra against comprehensive protein databases, while quantification methods assess protein abundance levels. Statistical analysis is employed to assess the significance of identified proteins and their differential expression across conditions. Advanced analysis techniques, including protein network analysis and pathway enrichment analysis, provide insights into protein interactions, functional relationships and biological pathways, facilitating a deeper understanding of complex proteomic data.
Standardization and Reproducibility
Establishing standardization and reproducibility are challenges in proteomics to ensure reliable and comparable results across different laboratories and platforms. It’s important to have standardized protocols and quality control measures to minimize experimental variability. Robust sample preparation methods, instrument performance validation and data acquisition protocols must be implemented to achieve reproducible results. Using reference materials and quality control samples allows the researchers to assess and monitor measurement accuracy and precision.
Dynamic Range and Sensitivity
The wide dynamic range of protein abundance in biological samples poses difficulties in detecting low-abundance proteins, as they can be overshadowed by high-abundance proteins. Additionally, the detection becomes saturated at high protein concentrations, limiting accurate quantification. To address these challenges, proteomic techniques strive to improve sensitivity and dynamic range through advancements in sample preparation, instrument sensitivity and data analysis methods. Enhanced sensitivity allows the detection of low-abundance proteins, while an extended dynamic range enables the accurate measurement of protein abundance across a wide concentration spectrum, providing deeper insights into the proteome’s complexity and dynamics.
Post-Translational Modifications
Detecting and analyzing post-translational modifications (PTMs) present challenges in proteomics due to their diverse nature and dynamic occurrence. PTMs play crucial roles in protein function, regulation and cellular processes. The characterization and quantification of PTMs require specialized enrichment techniques that selectively enrich modified peptides, sensitive detection methods capable of identifying and localizing specific modifications, and advanced data analysis tools to interpret PTM data accurately. Addressing the challenges associated with PTM protein regulation, signaling pathways and disease mechanisms provides valuable insights into cellular processes’ complexity.
Integration of Multiple Omics Data
Integrating proteomic data with other omics data, such as genomics and transcriptomics, presents some critical challenges. These challenges include data heterogeneity, different data formats and the need for standardized formats and data repositories. Integration methods and tools enable the correlation of protein abundance with gene expression, DNA variations and other molecular features, providing a more comprehensive understanding of cellular processes. By integrating multiple omics datasets, researchers can identify potential biomarkers, uncover functional relationships and gain deeper insights into complex biological systems.
Proteomics technologies
Proteomics technologies encompass a range of powerful tools for protein analysis. Two-dimensional polyacrylamide gel electrophoresis (2D-PAGE) separates proteins based on charge and size, enabling their visualization and comparison. Liquid chromatography (LC) techniques, including high-performance liquid chromatography (HPLC), aid in protein fractionation and purification. Isotope-coded affinity tag (ICAT) labeling allows for the quantitative analysis of proteins by labeling different protein samples with isotopic tags. Mass spectrometry is a cornerstone technology in proteomics, enabling the identification, characterization and quantification of proteins.
Two-Dimensional Polyacrylamide Gel Electrophoresis
2D-PAGE is a powerful technique used in proteomics for protein separation and analysis. The technique involves two key steps: isoelectric focusing (IEF) and sodium dodecyl sulfate-polyacrylamide gel electrophoresis (SDS-PAGE). In IEF, proteins are separated based on their isoelectric points, forming a pH gradient on a gel. This is followed by SDS-PAGE, where proteins are separated based on their molecular weights. 2D-PAGE allows the visualization of complex protein mixtures, enabling protein profiling, identification of differentially expressed proteins, and separation of protein complexes. It has been widely used in various fields, including disease biomarker discovery, drug target identification and studying protein-protein interactions.
If you are looking to perform protein gel electrophoresis and want equipment or products for the process, here is a wide-range of high-quality products and equipment for protein gel electrophoresis at Avantor. From precast gels to power supplies, we have you covered.
Liquid Chromatography
LC is a versatile technique extensively employed in proteomics for protein separation and purification. LC utilizes a stationary phase and a mobile phase to separate and elute proteins based on their physicochemical properties. Different modes of chromatography, such as reverse-phase, ion exchange and size exclusion, offer distinct separation mechanisms for proteins.
Additionally, LC plays a crucial role in protein fractionation that helps separate complex protein mixtures into individual components or protein subsets. It is particularly useful in separating peptides prior to mass spectrometry analysis, enhancing detection sensitivity and reducing sample complexity.
That said, you can explore our comprehensive range of complete HPLC systems at Avantor. From pumps and detectors to columns and software, we offer everything you need for efficient and reliable chromatographic separations. Furthermore, you can also avail the products for sample preparation for liquid chromatography (HPLC & UHPLC).
Isotope-Coded Affinity Tag Labeling
ICAT labeling is a technique used in quantitative proteomics to compare and quantify protein abundance. The principle of ICAT involves selectively labeling proteins with isotopically distinct tags, usually incorporating stable isotopes such as deuterium or carbon-13. This labeling allows for the differentiation and comparison of protein samples through mass spectrometry analysis. The steps in ICAT labeling include protein extraction, labeling with the isotopically labeled tags, enzymatic digestion and subsequent mass spectrometry analysis for quantification.
Mass Spectrometry
MS is a key analytical technique used in proteomics for protein identification and characterization. It involves the ionization of proteins, separation based on their mass-to-charge ratio, and detection of ionized molecules. Various ionization techniques, such as ESI and MALDI, are employed in MS-based proteomics.
Mass analyzers, including TOF, quadrupole, and Orbitrap, are used to measure the mass-to-charge ratio and obtain accurate mass information. Data analysis methods, such as database searching and spectral matching algorithms, are applied to identify proteins from the mass spectra.
You can benefit from mass spectrometry for your analytical needs with our cutting-edge range of mass spectrometers at Avantor. From high-resolution instruments to versatile ionization techniques, we offer advanced solutions for precise molecular analysis.
The future of proteomics
The future of proteomics holds great promise, with next-generation proteomics poised to provide an integrative view of proteome dynamics, encompassing not only protein expression levels but also their post-translational modifications, interactions and spatial organization within cells and tissues. This holistic approach will enable a deeper understanding of the functional implications of the proteome in various biological processes and disease states.
Technological improvements are expected to play a pivotal role in shaping the future of proteomics. These include advancements in mass spectrometry instrumentation, such as higher resolution and sensitivity, faster data acquisition rates and improved capabilities for multiplexed and targeted protein analysis. Finally, integration with other omics disciplines, such as genomics, transcriptomics and metabolomics, will be a key aspect of future proteomics research.
Conclusion
Proteomics is a powerful field of study that investigates the complex world of proteins within organisms. It plays a crucial role in uncovering protein functions, understanding disease mechanisms and advancing personalized medicine. This field of study finds practical applications in biomarker discovery, drug development and systems biology analysis. However, challenges like sample complexity, data analysis and standardization persist. Despite these lingering challenges, the future of proteomics looks promising, with advancements in next-generation techniques, improved technologies and integration with other omics disciplines.
You can learn more about the world of proteomics with Avantor comprehensive range of tools, equipment and resources. Whether you need sample preparation kits, mass spectrometers or bioinformatics solutions, we have you covered.